Everybody knows that lithium-ion batteries, used in cell phones, computers, etc., gradually lose capacity and eventually fail. This loss of capacity is primarily due to a layer known as the SEI1, which forms between one of the battery’s electrodes and the electrolyte (see Figure). This layer already appears after the first battery charge/discharge cycle, and grows over time, consuming lithium ions. The process is irreversible and therefore detrimental to battery capacity [1].
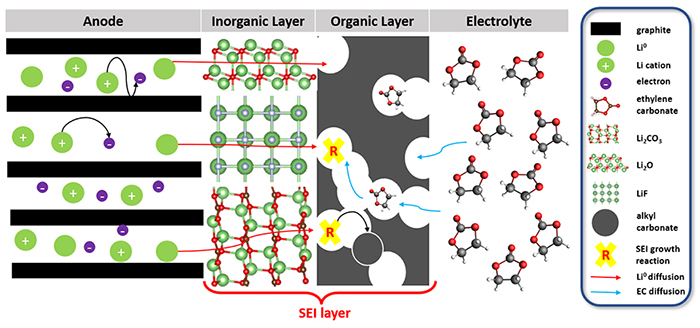
While the chemical composition of this SEI layer can be determined through experimental analysis, other aspects concerning its formation, such as the thermodynamic conditions of its appearance and its growth kinetics, are more advantageously studied using theoretical approaches, such as molecular modeling. Methods based on quantum chemistry (QM) are well adapted to this need, since they explicitly take into account electrons, which play a key role in the solvent (electrolyte component) reduction reactions at the negative electrode interface, and it is this set of reactions that initiates and fuels the formation of the SEI layer.
However, QM based methods require too many IT resources to be applied to a system the size of a battery. A multi-scale approach therefore appears to be a suitable alternative, enabling a detailed description of the chemical reactions involved in the solvent degradation over periods of time ranging from the picosecond to a second (or more). The QM approach can then be extended by use of the Kinetic Monte Carlo (kMC2) simulations.
The first stage of this PhD research involved establishing a database of the chemical reactions involved, with their reaction energies and their activation energies [2], measurements that have a direct influence on the reaction rate. These energies were calculated with methods based on DFT3.
The kMC simulations subsequently conducted showed that salts such as Li2CO3 ou Li2O, resulting from electrolyte reduction, played a significant role in the formation of the SEI [2]. These salts promoted the appearance of various organic degradation species, whose presence corroborated analytical observations and result in a multi-layered SEI structure.
kMC simulations were also used to predict the loss of battery capacity as a function of the composition of the SEI layer initially formed (Figure 2). It turns out that in the absence of Li2CO3 ou Li2O, this capacity reduction is non-linear, and that it is slower in their presence. These results tally with other experimental and theoretical studies [3] and encourage further research taking into account the appearance of other salts resulting from the degradation reactions, such as LiF. In the future, the aim is to expand the reference library of degradation reactions that can take place in the presence of these salts, and to use methods that require fewer CPU resources than DFT.
1- Solid Electrolyte Interphase.
2- Method used to simulate the behavior of systems evolving as a function of a master equation, using kinetic data relating to elementary chemical reactions.
3- Density Functional Theory.
* Thesis title: Gaining a better understanding of the formation and growth of the Solid Electrolyte Interphase in Li-ion batteries via a molecular modeling approach.
References:
-
A. Wang, S. Kadam, H. Li, S. Shi, Y. Qi, Review on modeling of the anode solid electrolyte interphase (SEI) for lithium-ion batteries, NPJ Comput. Mater. 4 (2018) 359.
>> https://doi.org/10.1038/s41524-018-0064-0
-
Mohammed Bin Jassar, Carine Michel, Sara Abada, Theodorus de Bruin, Sylvain Tant, Carlos Nieto-Draghi, Stephan N. Steinmann. A joint DFT-kMC study to model ethylene carbonate decomposition reactions: SEI formation, growth, and capacity loss during calendar aging of Li-metal batteries, ACS Appl. Energy Mater.
>> https://doi.org/10.1021/acsaem.3c00372
-
Kolzenberg, L. von; Latz, A.; Horstmann, B. Solid-Electrolyte Interphase During Battery Cycling: Theory of Growth Regimes. ChemSusChem 2020.
>> https://doi.org/10.1002/cssc.202000867
Scientific Contacts: carlos.nieto@ifpen.fr ; theodorus.de-bruin@ifpen.fr